Nuclear Magnetic Resonance (NMR) spectroscopy is an analytical technique used to determine the molecular structure and chemical composition of samples. Its operating principle involves analyzing the interaction of rotating nuclei in a strong magnetic field. In NMR spectroscopy, a fixed external magnetic field causes certain atomic nuclei within a molecule to selectively absorb radiofrequency energy. The absorption of energy induces a change in nuclear spin, which can be observed in the nuclear magnetic resonance spectrum.
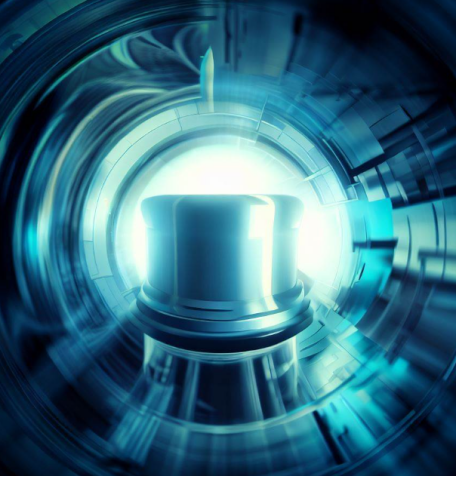
Applications of Nuclear Magnetic Resonance Spectroscopy
Nuclear Magnetic Resonance spectroscopy is a non-destructive and non-invasive technique employed for elucidating molecular structures and dynamics. NMR finds diverse applications across various fields and industries, including:
In biology, NMR is used to study large molecules such as proteins, lipids, and nucleic acids. NMR-active nuclei such as 13C, 1H, 15N, 31P, 23Na, and 19F are employed to understand biochemical pathways involving amino acids, lipids, and carbohydrate metabolism.
In chemistry, NMR is widely utilized for qualitative and quantitative analysis, monitoring reactions, identifying structures, and assessing purity.
In polymer science, it is used to analyze monomer ratios, molecular weights, structural regularity, branching, chain length, and end groups.
In the pharmaceutical industry, NMR is employed to determine the purity and quantity of active ingredients, excipients, and impurities in drugs.
In the petroleum industry, it is used to evaluate the hydrocarbon content of crude oil and its products.
In medicine, Magnetic Resonance Imaging (MRI) is an application of NMR used for soft tissue analysis to identify damaged or diseased tissues.
Principles of Nuclear Magnetic Resonance Spectroscopy
Nuclear spin is related to the composition of an element's nucleus. Nuclei with an even number of both protons and neutrons have zero nuclear spin and are not amenable to NMR (e.g., 4He, 12C, 16O). Nuclei with an odd number of protons and/or neutrons exhibit nuclear spin and can undergo NMR (e.g., 1H, 2H, 14N, 17O). These nuclei behave like miniature spinning magnets and can interact with an external magnetic field. The spinning nuclei also generate their own magnetic field, which can interact with other nuclei possessing spin.
An NMR instrument measures the interaction of nuclear spin states under the influence of a strong magnetic field. The magnetic field causes nuclei to precess (rotate) akin to spinning tops. A precessing nucleus selectively absorbs energy from radiofrequency waves when the frequency of the precessing nuclei matches the low-frequency of externally applied radiofrequency waves. This phenomenon is termed "resonance," hence the name "nuclear magnetic resonance." Resonance can be achieved by either tuning the frequency of the nuclei to match the fixed radiofrequency or by adjusting the frequency of the radio waves to match that of the nuclei.
During NMR, the applied magnetic field excites nuclei at different energy levels with distinct magnetic moments. After absorbing specific radiofrequency energy, the excited-state nuclei return to lower energy states by transferring energy to their surroundings. Energy transfer to other atoms or the solvent is termed "spin-lattice relaxation," while transfer to neighboring nuclei at the same energy level is referred to as "spin-spin relaxation." These two relaxation processes are characterized by time constants: spin-lattice relaxation time (T1) and spin-spin relaxation time (T2), which collectively determine the resulting NMR spectrum.
Characteristics of an NMR Spectrum
An NMR spectrum is a plot of applied radiofrequency against absorption. The position on the plot where the nuclei absorb is known as the chemical shift. Chemical shift is influenced by the electron density around the nucleus. When a nucleus is surrounded by high electron density, it becomes shielded from the external magnetic field, causing signals to shift upfield on the NMR spectrum. Conversely, when a nucleus is surrounded by electronegative atoms, which reduce electron density around the nucleus, a "deshielding" effect occurs, shifting the signal downfield on the NMR spectrum. Additionally, the spin of neighboring nuclei can impact the signals observed on an NMR spectrum, potentially resulting in signal splitting, known as "spin-spin coupling."